
Negative forces are measured between 0 and >1 G.
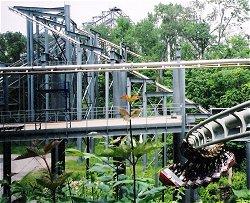
Negative G’s are considered to be the most fun G Force but also the most dangerous. Negative G’s are typically experienced when a train crests a hill at speed, or suddenly dips sharply downwards. Positive G is when riders feel heavier from pressure bearing down, as if they are being pushed down into the seat. For example, on Oblivion at Alton Towers, when the train pulls back up from the vertical drop, a massive 4.5G is exerted on riders for a brief moment. Positive G forces typically occur on banked turns or when the train pulls up a hill after going down a drop. The sharpness of this change of direction determines the positive G. Positive G-Force occurs at the bottom of a drop when the train wants to continue moving in the same direction, but the track forces it in a different direction. Floater Airtime is a smoother sensation where the rider feels they are weightless.
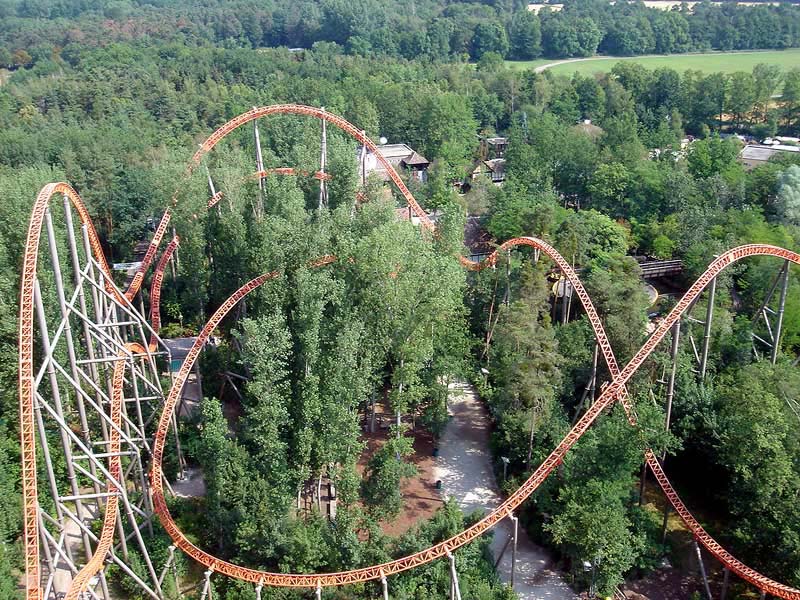
Ejector Airtime is where the rider feels they are being quickly ejected from the coaster. G Forces create the airtime that riders experience. G Forces vary depending on the element and the entry/exit into and out of it some elements are more intense than others. In simpler terms, if a rider person weighs 100 kg while standing still, they would momentarily weigh 400 kg at the 4G point of the ride. If a theme park says a coaster rider will experience, for example, 4G, the rider will briefly experience four times the force of gravity. Everybody experiences 1G in everyday life, the regular force of gravity when still. G stands for Gravity and the number in front of it represents how many times the force of gravity is felt at a particular point. These forces are known as G-Forces or “G’s”. Several forces are felt when riding a coaster, and keeping these within safe values is a vital skill for coaster designers. Providing the element after the first drop is low enough to take the weight of the train into consideration, this is the perfect situation allowing the coaster to continue to the next element. Situation 3: An Element of Less Height than the First Drop The train would only make it about 3/4 of the way up the second hill before it rolls back down. Even though, theoretically, the train has the kinetic energy to get up the same size hill as the first drop, some kinetic energy would be lost yet again due to friction and air resistance. In this situation, when the train accelerates down the first drop and climbs up the second element, it would roll back. This would be another dull coaster, but this would make the news as it is destined to get stuck. And it would be a very boring coaster! Situation 2: An Element of Equal Height to the First Drop Train will stop moving without kinetic energy.

However, in the real world, forces such air resistance and friction between the wheels and the track dissipate the kinetic energy. If the track after the first drop was completely flat and straight, the kinetic energy would, theoretically, allow the train to continue moving forever, as energy does not disappear. Coaster designers then have to consider what happens after the first drop. The calculation between the lift hill and the drop heights have to be precise, otherwise the train will not gather enough potential and kinetic energy to complete the circuit. The kinetic energy at the bottom of the drop determines how far the coaster train can travel along the track and through inversions, banked turns and airtime hills. The coaster rails control the angle of descent meaning the steeper the first drop, the greater the kinetic energy. All objects falling to the ground seek the fastest way down which is typically straight down. The greater the potential energy in the train gathered during the lift hill climb, the more kinetic energy the train will have at the bottom of the drop. A transfer of Potential Energy to Kinetic Energy occurs when the coaster train leaves the top of the lift hill and powers down the first drop. When an object falls back to Earth, it gathers Kinetic Energy.
